Quantum computers function in a fundamentally distinct manner compared to traditional digital computers. Quantum computing relies on the principles of quantum mechanics, leveraging phenomena that occur at the atomic, electron, and photon levels. The process entails encoding and manipulating information by managing quantum states, depending on the distinctive quantum properties of superposition and entanglement.
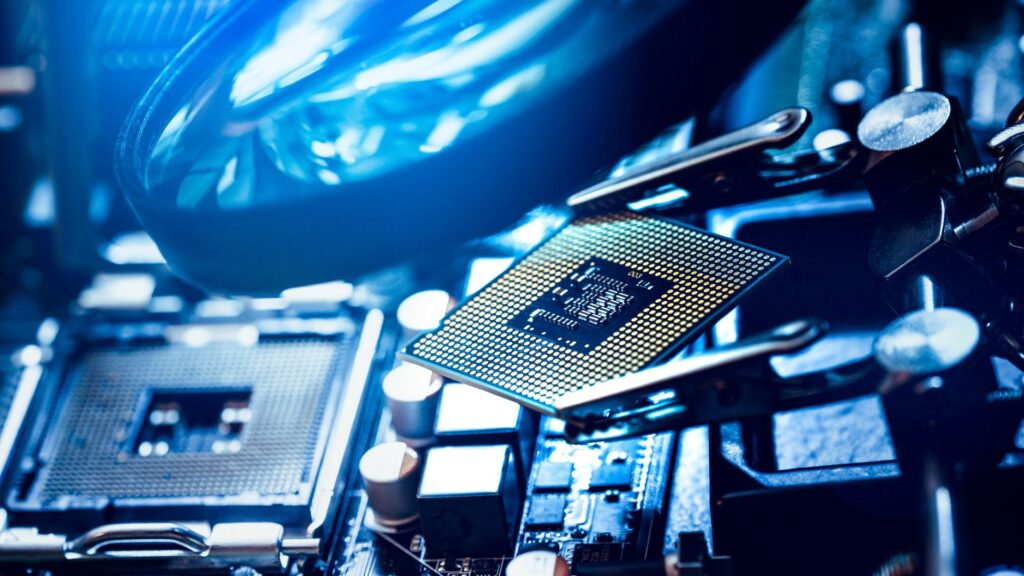
Qubits
In traditional computing, data is expressed using binary digits or ‘bits,’ which are fundamental units of information represented as either ‘0’ or ‘1.’ In quantum computing, the equivalent unit is a quantum bit or ‘qubit.’ Qubits can exist in a unique state as either ‘0’ or ‘1,’ or in a simultaneous combination of both due to superposition. Multiple qubits can form a register, and through quantum entanglement, they can become correlated in a way that goes beyond the capabilities of the classical world. Quantum entanglement is a subtle and powerful phenomenon. The interplay of superposition and entanglement allows a quantum register to encode information in a vast number of states simultaneously. This unique feature is pivotal in harnessing the computational power of a quantum computer, enabling it to perform calculations for tasks that are currently impractical for even the most powerful conventional supercomputers.
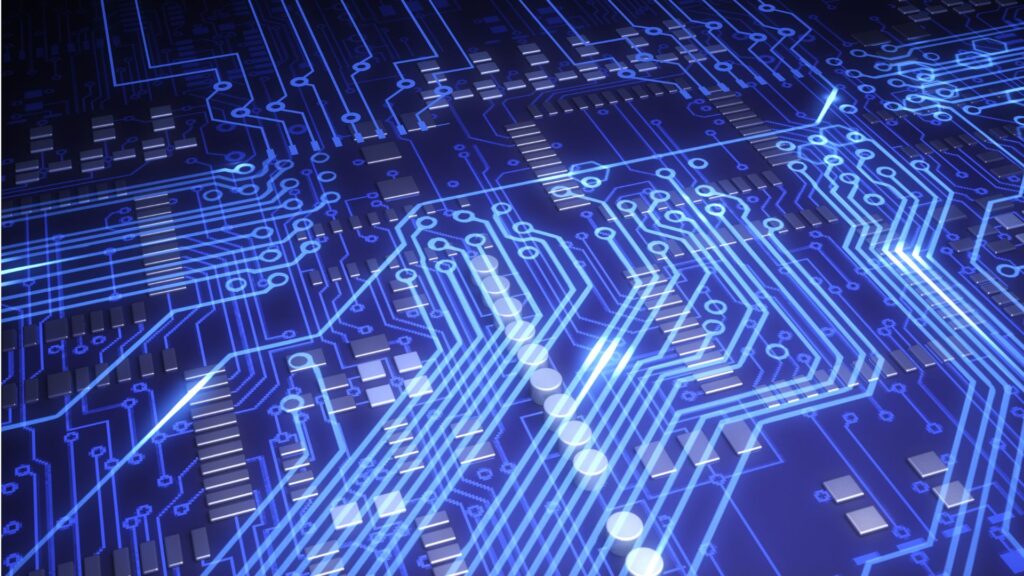
Full stack & architecture
Quantum computers can adopt various architectures, built upon alternative designs and constructed through different methods. Similar to classical computers, the construction of a quantum computer involves multiple layers of hardware and software, spanning from the processor to the user interface. This entirety of layers is commonly referred to as the full stack.
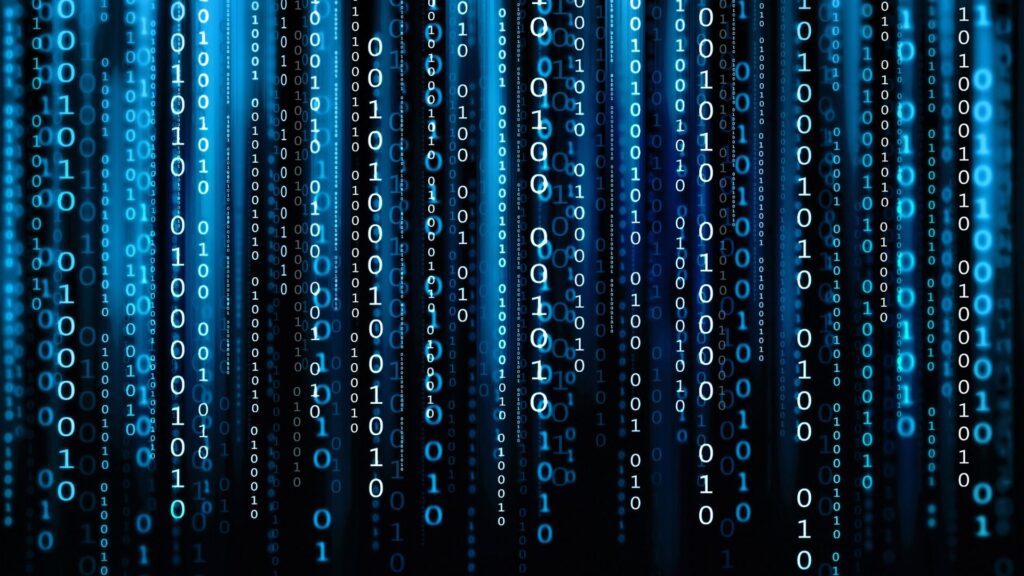
Applications
The advent of quantum computing is anticipated to have a substantial impact across various sectors, leading to enhancements in efficiency, productivity, and competitiveness, along with the development of novel products and services. Some promising early applications encompass the modeling and analysis of more intricate molecules to expedite the discovery of new materials and pharmaceuticals, the optimization of complex planning and scheduling in logistics, improvements in the design and manufacturing processes in sectors like aerospace and automotive, and the optimization of networks including traffic routing, telecommunications, and energy distribution.
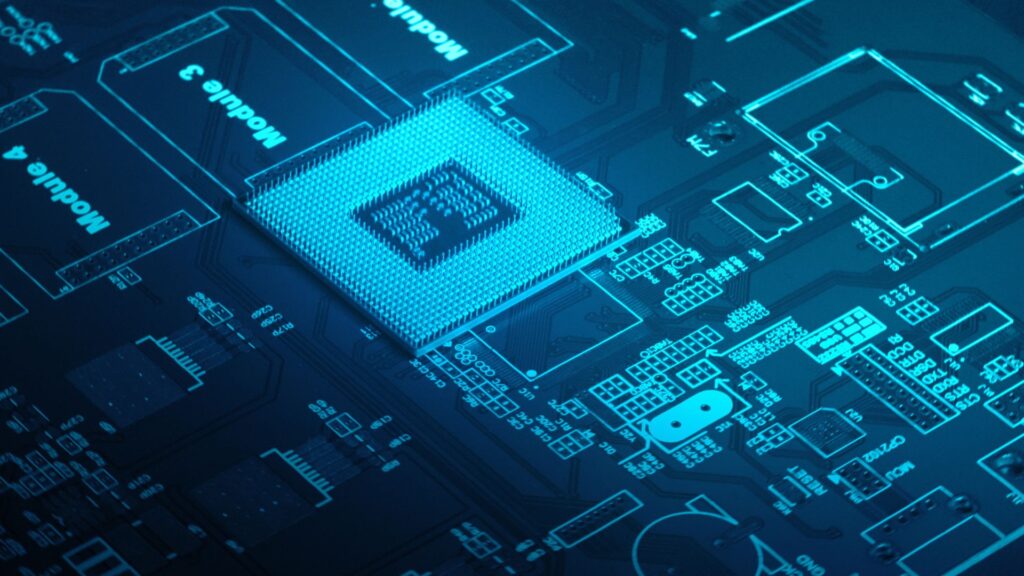
Technical challenges
There are several potential hardware architectures for building quantum computers, each with its own advantages and at different stages of technological maturity. The common characteristic among them is that physical qubits are inherently delicate, requiring precise control and protection from the external environment. It is crucial for the states to be long-lived, and operations on them need to be high-fidelity to prevent the accumulation of errors, which would necessitate additional computational resources for error correction.
Researchers are exploring architectures that may require limited or even no error correction. While the field has made continuous technical advancements, there are substantial engineering challenges in scaling up to larger numbers of qubits with low noise. Understanding the merits of different hardware architectures is essential as quantum computers are scaled. A significant breakthrough would involve constructing and demonstrating quantum computers that exhibit enhanced performance across various tasks.
In the short term, addressing some of these challenges involves developing NISQ (noisy intermediate-scale quantum) machines. These machines offer a potential pathway to achieving a general-purpose quantum computer in the future.
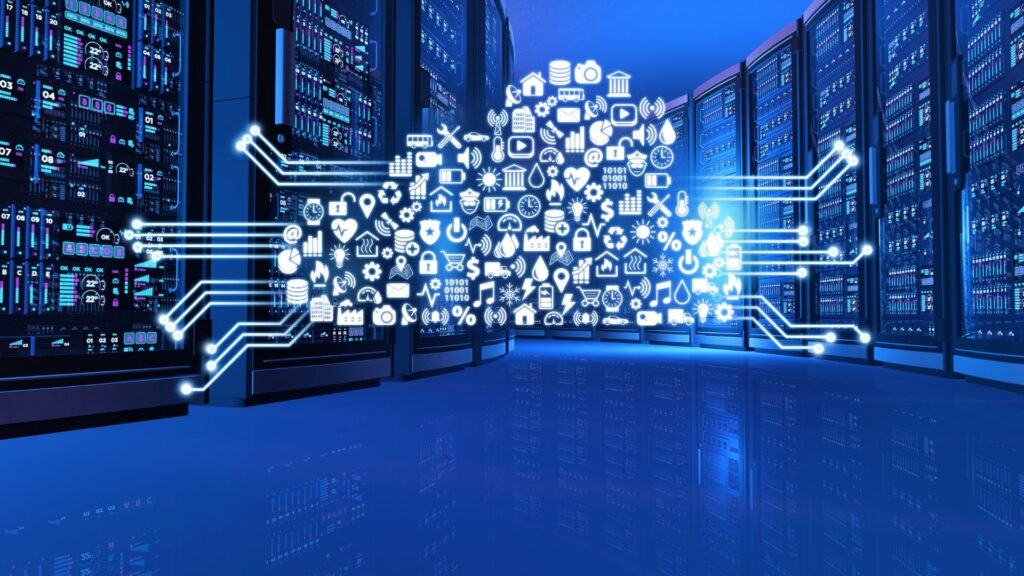